Hybrid Linac-MRI Technology Developed to Image and Treat Tumors
By MedImaging International staff writers Posted on 14 Aug 2009 |
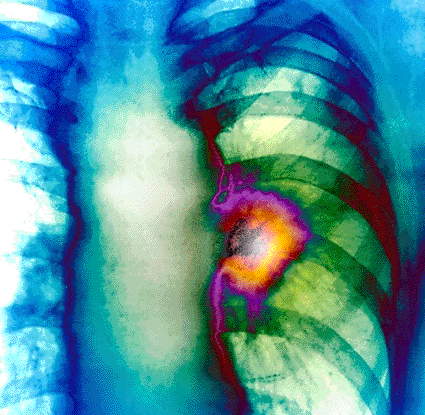
Image: Colored X-ray of the left lung in a patient with lung cancer (Photo courtesy of Zephyr / SPL).
Canadian scientists are developing a new technology that integrates two existing medical devices--medical linear accelerators, linacs, which produce powerful X-rays for treating cancer, and magnetic resonance imaging (MRI) systems, which are widely used to image tumors in the human body.
The proposed hybrid Linac-MR system promises to help clinicians treat specific types of cancer by allowing them to accurately monitor moving tumors in people's lungs and other soft tissues such as the liver or prostate in real time while the radiation treatment is ongoing. Though the new technology is not yet available in the clinic, the scientists, from the University of Alberta's Cross Cancer Institute (Edmonton, AB, Canada), have now demonstrated its feasibility for the near future.
In related research, a group from Stanford University (Stanford, CA, USA) is determining the specifications for how the new technology can be used. Both groups discussed their latest findings at the 51st meeting of the American Association of Physicists in Medicine (AAPM), July 26-30, 2009, in Anaheim, CA, USA.
The success of modern cancer radiotherapy often depends on how well radiation oncologists and medical physicists can determine the exact location and shape of a tumor. When doctors plan radiotherapy treatment for their patients, they first define the outline of target tumors by collecting high-resolution three-dimensional (3D) images. The better the outline of a tumor is defined, the more precisely clinicians can irradiate it, and the more successfully the cancerous cells inside are killed while sparing the surrounding healthy tissue.
Imaging techniques have improved to the point that doctors can now define the edges of many tumors to within a fraction of an inch. During treatment, however, many tumors will move. Tumors in the lungs, for instance, can move up to an inch or more as a person breathes, complicating treatment. Therefore, traditionally, radiation is delivered to a slightly larger area surrounding the tumor to ensure that the tumor is always in the beam's sight. This means, however, that adjacent normal tissue as well as critical organs such as the heart and spinal cord may receive harmful radiation dose.
In recent years, image-guided radiation treatment (IGRT) has emerged as a technique for following tumors as they move. With IGRT, clinicians typically use implanted markers to localize the tumor or X-rays to generate computed tomography (CT) images of the patient just prior to treatment to determine the tumor position on that day and adjust the patient position to place the tumor to coincide with the high radiation dose.
According to Dr. B. Gino Fallone, the director of medical physics at the University of Alberta's Cross Cancer Institute, image-guided radiotherapy is simply the latest sophisticated way of doing what radiologists have always done. "Track it and treat it,” he stated. "That's been the goal of radiation oncology for 50 years.”
While existing IGRT techniques are effective, they are limited because they do not give true image-based guidance of the entire volume of the tumor, according to Dr. Amit Sawant, an instructor in the department of radiation oncology at Stanford University. Instead of imaging the whole anatomic volume containing the tumor, markers and seeds provide a few points in space that doctors can follow--something Dr. Sawant refers to as "faith-based radiation delivery.”
While CT provides 3D images, it is frequently difficult to distinguish tumors from normal tissues with CT scans. MRI provides superior distinction between normal and cancerous tissues, but the technology has not been available to place an MR scanner in the treatment room. A more robust way to guide radiotherapy would be to image the entire tumor continuously and adjust the radiation beams accordingly. Dr. Fallone and his colleagues are evaluating a prototype Linac-MR system they built to do just that.
Linacs (linear particle accelerators) are devices that use radio waves to accelerate electrons to high speeds and crash them into a solid metal target--typically tungsten--producing high-energy X-rays in the collision. These high-energy X-rays destroy cancerous cells by causing irreparable damage to the cells' DNA, MRI systems, familiar because of their ubiquity in modern hospitals, are very good at imaging soft tissue and would be an ideal technology for combining with Linacs because most cancers occur in soft tissue.
The problem is making MRIs and Linacs work together. Normally, each one would interfere with the other. Linac systems emit radio waves, which interfere with MRI hardware--so much so that most hospital MRI units are placed in shielded rooms that specifically block radio waves. At the same time, MRI devices employ strong magnets that can interfere with Linac systems.
Dr. Fallone and his colleagues have managed to build a prototype linac-MR device that overcomes the obstacles to integrating the two technologies. It is the first working system to do so. They have a specialized design that shields the radio frequency waves and magnetic fields. In December 2008, they performed experiments with the system to demonstrate that it works by taking MRI test images with the linac on and off to demonstrate that the interference is eliminated. A working, clinically ready system is not here yet, but Dr. Fallone believes that it will be in about five years.
In related research, Dr. Sawant and his Stanford colleagues Drs. Kim Butts Pauly and Paul Keall have been working out the technical details of just how a hybrid MRI+linac system could be used to achieve real-time image guidance. Typical imaging times for MRI range from several seconds to a few minutes per image. Dr. Sawant noted that their goal is to go at least 10 times faster. They are developing imaging specifications for a system to accurately image the entire volume of a tumor in real time, about three times per second.
The key to implementing this technology may be to lower the magnetic field of the magnetic resonance used to image a tumor. Powerful hospital MR instruments employ large magnets with strong magnetic fields because these will give the best quality images. Better quality images help doctors spot tumors, and when someone with cancer is diagnosed, doctors prefer to have images that are as clear as possible, for accurate classification of the tumor. Integrating such strong magnets with linear accelerators is expensive and can be technically quite challenging.
During therapy, however, the images need not be the highest quality in order to track the tumors. Doctors simply need to be able to see the tumor boundaries so that they can make adjustments as the treatment proceeds. At the meeting, Dr. Sawant and his colleagues described the results of their studies, which indicate the feasibility of such rapid imaging for hybrid MRI+linac systems in order to follow tumors in real time.
Related Links:
University of Alberta's Cross Cancer Institute
Stanford University
The proposed hybrid Linac-MR system promises to help clinicians treat specific types of cancer by allowing them to accurately monitor moving tumors in people's lungs and other soft tissues such as the liver or prostate in real time while the radiation treatment is ongoing. Though the new technology is not yet available in the clinic, the scientists, from the University of Alberta's Cross Cancer Institute (Edmonton, AB, Canada), have now demonstrated its feasibility for the near future.
In related research, a group from Stanford University (Stanford, CA, USA) is determining the specifications for how the new technology can be used. Both groups discussed their latest findings at the 51st meeting of the American Association of Physicists in Medicine (AAPM), July 26-30, 2009, in Anaheim, CA, USA.
The success of modern cancer radiotherapy often depends on how well radiation oncologists and medical physicists can determine the exact location and shape of a tumor. When doctors plan radiotherapy treatment for their patients, they first define the outline of target tumors by collecting high-resolution three-dimensional (3D) images. The better the outline of a tumor is defined, the more precisely clinicians can irradiate it, and the more successfully the cancerous cells inside are killed while sparing the surrounding healthy tissue.
Imaging techniques have improved to the point that doctors can now define the edges of many tumors to within a fraction of an inch. During treatment, however, many tumors will move. Tumors in the lungs, for instance, can move up to an inch or more as a person breathes, complicating treatment. Therefore, traditionally, radiation is delivered to a slightly larger area surrounding the tumor to ensure that the tumor is always in the beam's sight. This means, however, that adjacent normal tissue as well as critical organs such as the heart and spinal cord may receive harmful radiation dose.
In recent years, image-guided radiation treatment (IGRT) has emerged as a technique for following tumors as they move. With IGRT, clinicians typically use implanted markers to localize the tumor or X-rays to generate computed tomography (CT) images of the patient just prior to treatment to determine the tumor position on that day and adjust the patient position to place the tumor to coincide with the high radiation dose.
According to Dr. B. Gino Fallone, the director of medical physics at the University of Alberta's Cross Cancer Institute, image-guided radiotherapy is simply the latest sophisticated way of doing what radiologists have always done. "Track it and treat it,” he stated. "That's been the goal of radiation oncology for 50 years.”
While existing IGRT techniques are effective, they are limited because they do not give true image-based guidance of the entire volume of the tumor, according to Dr. Amit Sawant, an instructor in the department of radiation oncology at Stanford University. Instead of imaging the whole anatomic volume containing the tumor, markers and seeds provide a few points in space that doctors can follow--something Dr. Sawant refers to as "faith-based radiation delivery.”
While CT provides 3D images, it is frequently difficult to distinguish tumors from normal tissues with CT scans. MRI provides superior distinction between normal and cancerous tissues, but the technology has not been available to place an MR scanner in the treatment room. A more robust way to guide radiotherapy would be to image the entire tumor continuously and adjust the radiation beams accordingly. Dr. Fallone and his colleagues are evaluating a prototype Linac-MR system they built to do just that.
Linacs (linear particle accelerators) are devices that use radio waves to accelerate electrons to high speeds and crash them into a solid metal target--typically tungsten--producing high-energy X-rays in the collision. These high-energy X-rays destroy cancerous cells by causing irreparable damage to the cells' DNA, MRI systems, familiar because of their ubiquity in modern hospitals, are very good at imaging soft tissue and would be an ideal technology for combining with Linacs because most cancers occur in soft tissue.
The problem is making MRIs and Linacs work together. Normally, each one would interfere with the other. Linac systems emit radio waves, which interfere with MRI hardware--so much so that most hospital MRI units are placed in shielded rooms that specifically block radio waves. At the same time, MRI devices employ strong magnets that can interfere with Linac systems.
Dr. Fallone and his colleagues have managed to build a prototype linac-MR device that overcomes the obstacles to integrating the two technologies. It is the first working system to do so. They have a specialized design that shields the radio frequency waves and magnetic fields. In December 2008, they performed experiments with the system to demonstrate that it works by taking MRI test images with the linac on and off to demonstrate that the interference is eliminated. A working, clinically ready system is not here yet, but Dr. Fallone believes that it will be in about five years.
In related research, Dr. Sawant and his Stanford colleagues Drs. Kim Butts Pauly and Paul Keall have been working out the technical details of just how a hybrid MRI+linac system could be used to achieve real-time image guidance. Typical imaging times for MRI range from several seconds to a few minutes per image. Dr. Sawant noted that their goal is to go at least 10 times faster. They are developing imaging specifications for a system to accurately image the entire volume of a tumor in real time, about three times per second.
The key to implementing this technology may be to lower the magnetic field of the magnetic resonance used to image a tumor. Powerful hospital MR instruments employ large magnets with strong magnetic fields because these will give the best quality images. Better quality images help doctors spot tumors, and when someone with cancer is diagnosed, doctors prefer to have images that are as clear as possible, for accurate classification of the tumor. Integrating such strong magnets with linear accelerators is expensive and can be technically quite challenging.
During therapy, however, the images need not be the highest quality in order to track the tumors. Doctors simply need to be able to see the tumor boundaries so that they can make adjustments as the treatment proceeds. At the meeting, Dr. Sawant and his colleagues described the results of their studies, which indicate the feasibility of such rapid imaging for hybrid MRI+linac systems in order to follow tumors in real time.
Related Links:
University of Alberta's Cross Cancer Institute
Stanford University
Latest MRI News
- AI Tool Tracks Effectiveness of Multiple Sclerosis Treatments Using Brain MRI Scans
- Ultra-Powerful MRI Scans Enable Life-Changing Surgery in Treatment-Resistant Epileptic Patients
- AI-Powered MRI Technology Improves Parkinson’s Diagnoses
- Biparametric MRI Combined with AI Enhances Detection of Clinically Significant Prostate Cancer
- First-Of-Its-Kind AI-Driven Brain Imaging Platform to Better Guide Stroke Treatment Options
- New Model Improves Comparison of MRIs Taken at Different Institutions
- Groundbreaking New Scanner Sees 'Previously Undetectable' Cancer Spread
- First-Of-Its-Kind Tool Analyzes MRI Scans to Measure Brain Aging
- AI-Enhanced MRI Images Make Cancerous Breast Tissue Glow
- AI Model Automatically Segments MRI Images
- New Research Supports Routine Brain MRI Screening in Asymptomatic Late-Stage Breast Cancer Patients
- Revolutionary Portable Device Performs Rapid MRI-Based Stroke Imaging at Patient's Bedside
- AI Predicts After-Effects of Brain Tumor Surgery from MRI Scans
- MRI-First Strategy for Prostate Cancer Detection Proven Safe
- First-Of-Its-Kind 10' x 48' Mobile MRI Scanner Transforms User and Patient Experience
- New Model Makes MRI More Accurate and Reliable
Channels
Radiography
view channel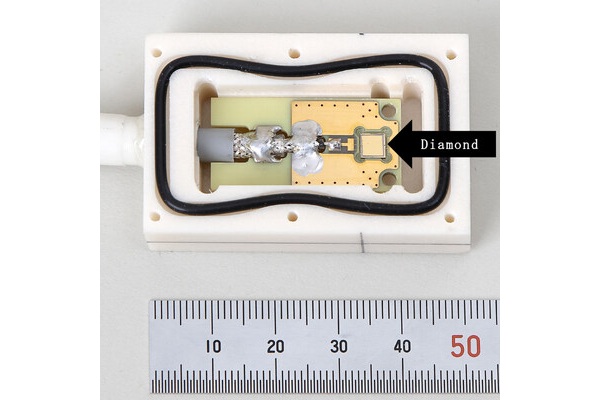
World's Largest Class Single Crystal Diamond Radiation Detector Opens New Possibilities for Diagnostic Imaging
Diamonds possess ideal physical properties for radiation detection, such as exceptional thermal and chemical stability along with a quick response time. Made of carbon with an atomic number of six, diamonds... Read more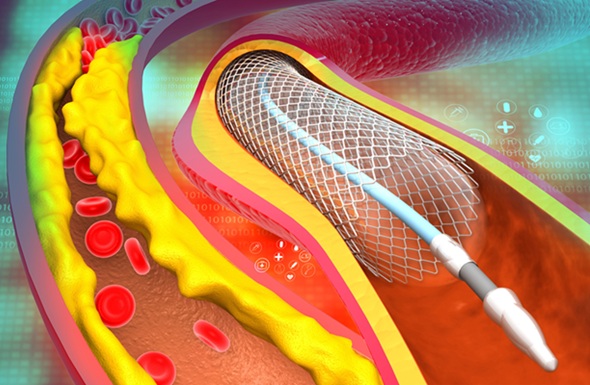
AI-Powered Imaging Technique Shows Promise in Evaluating Patients for PCI
Percutaneous coronary intervention (PCI), also known as coronary angioplasty, is a minimally invasive procedure where small metal tubes called stents are inserted into partially blocked coronary arteries... Read moreUltrasound
view channel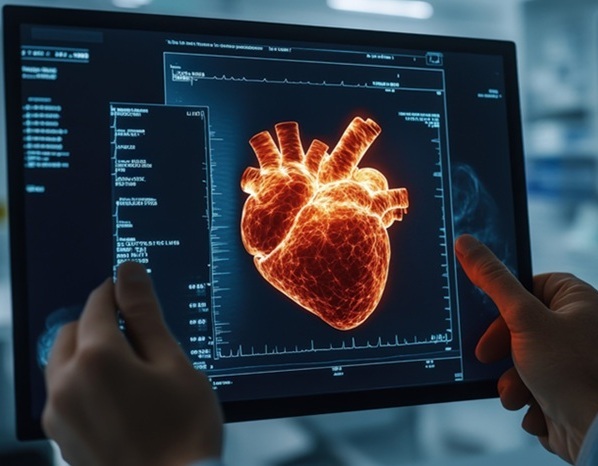
AI Identifies Heart Valve Disease from Common Imaging Test
Tricuspid regurgitation is a condition where the heart's tricuspid valve does not close completely during contraction, leading to backward blood flow, which can result in heart failure. A new artificial... Read more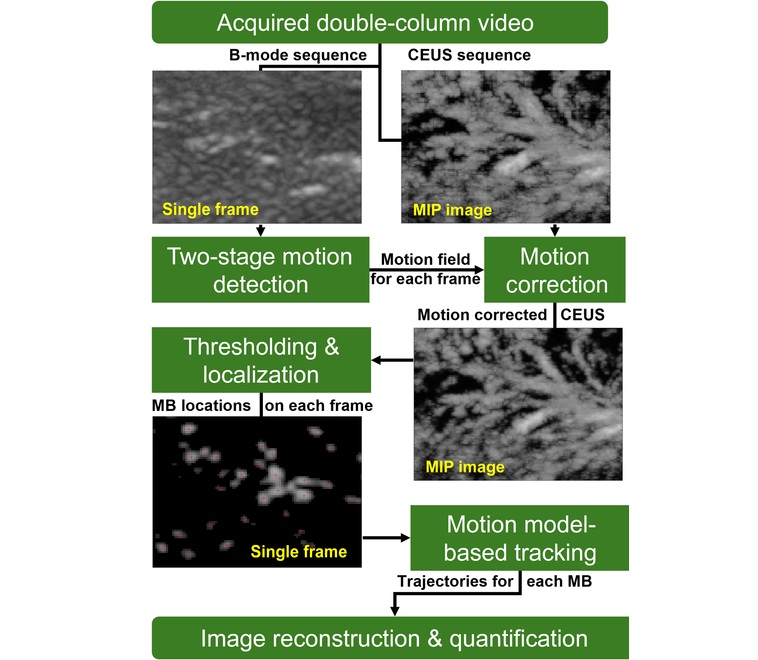
Novel Imaging Method Enables Early Diagnosis and Treatment Monitoring of Type 2 Diabetes
Type 2 diabetes is recognized as an autoimmune inflammatory disease, where chronic inflammation leads to alterations in pancreatic islet microvasculature, a key factor in β-cell dysfunction.... Read moreNuclear Medicine
view channel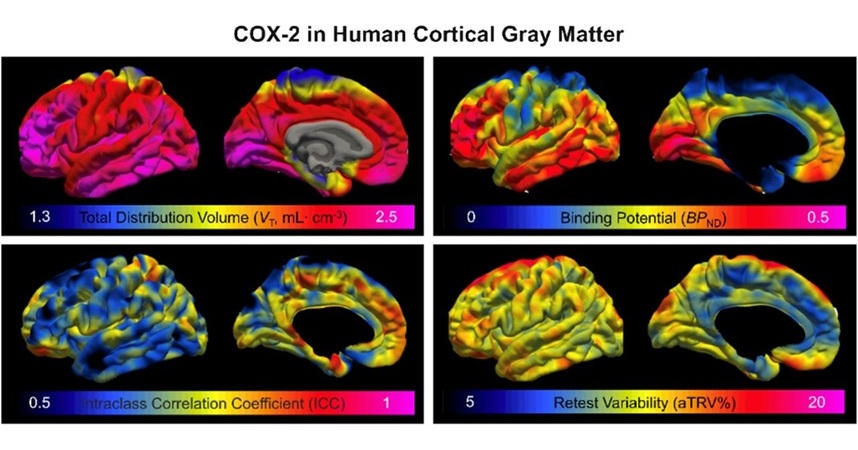
Novel PET Imaging Approach Offers Never-Before-Seen View of Neuroinflammation
COX-2, an enzyme that plays a key role in brain inflammation, can be significantly upregulated by inflammatory stimuli and neuroexcitation. Researchers suggest that COX-2 density in the brain could serve... Read more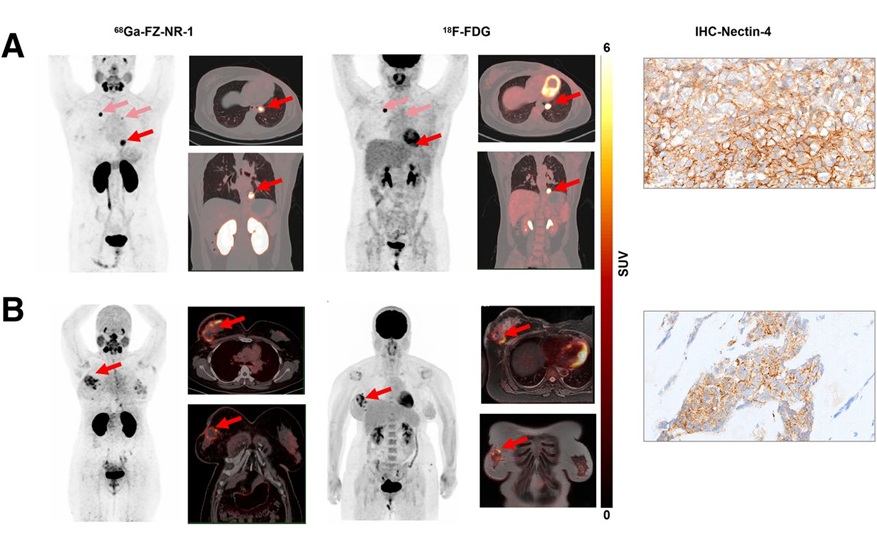
Novel Radiotracer Identifies Biomarker for Triple-Negative Breast Cancer
Triple-negative breast cancer (TNBC), which represents 15-20% of all breast cancer cases, is one of the most aggressive subtypes, with a five-year survival rate of about 40%. Due to its significant heterogeneity... Read moreGeneral/Advanced Imaging
view channel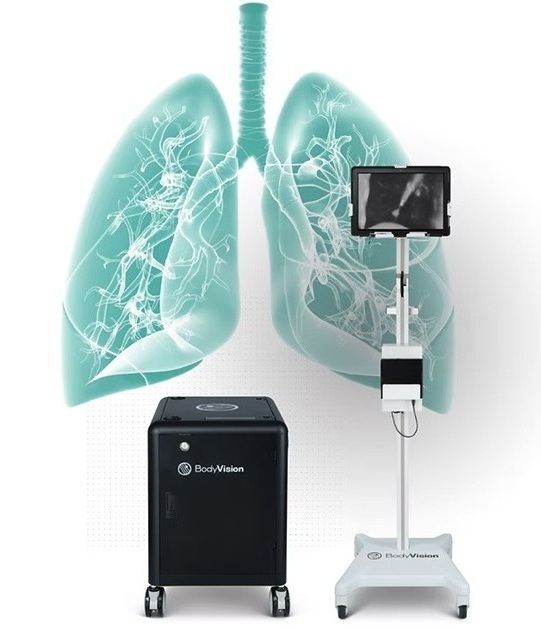
AI-Powered Imaging System Improves Lung Cancer Diagnosis
Given the need to detect lung cancer at earlier stages, there is an increasing need for a definitive diagnostic pathway for patients with suspicious pulmonary nodules. However, obtaining tissue samples... Read more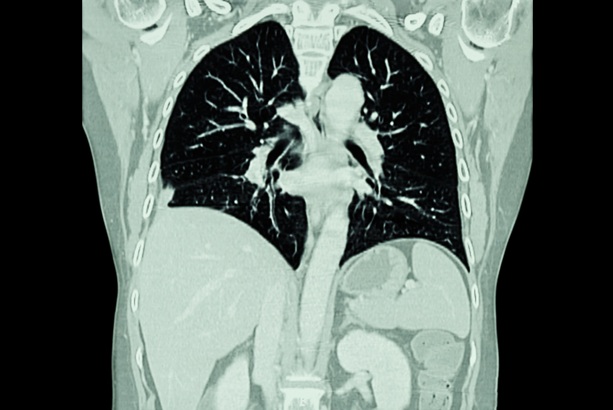
AI Model Significantly Enhances Low-Dose CT Capabilities
Lung cancer remains one of the most challenging diseases, making early diagnosis vital for effective treatment. Fortunately, advancements in artificial intelligence (AI) are revolutionizing lung cancer... Read moreImaging IT
view channel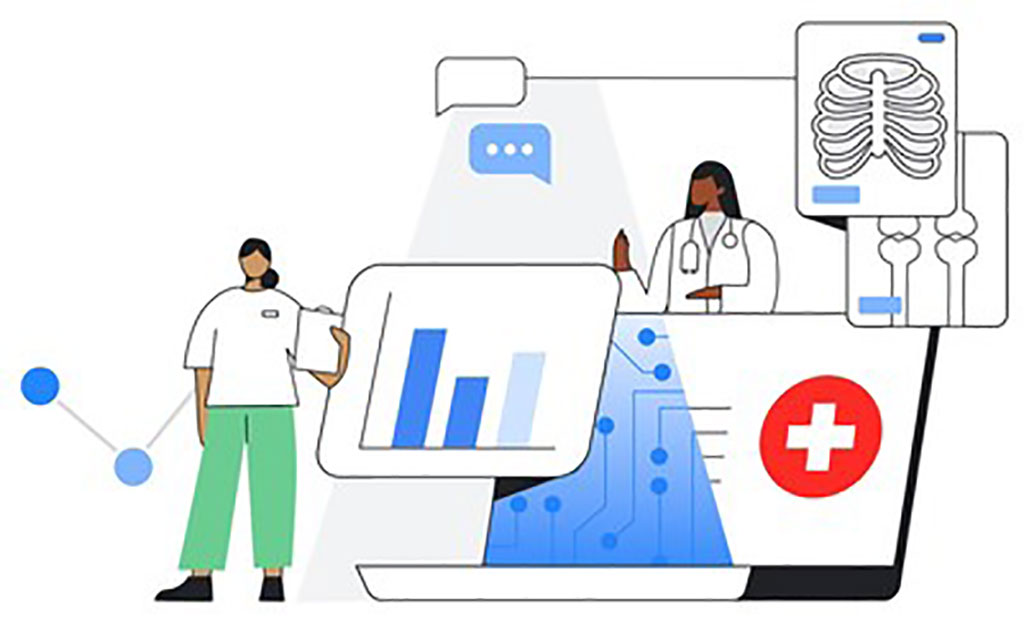
New Google Cloud Medical Imaging Suite Makes Imaging Healthcare Data More Accessible
Medical imaging is a critical tool used to diagnose patients, and there are billions of medical images scanned globally each year. Imaging data accounts for about 90% of all healthcare data1 and, until... Read more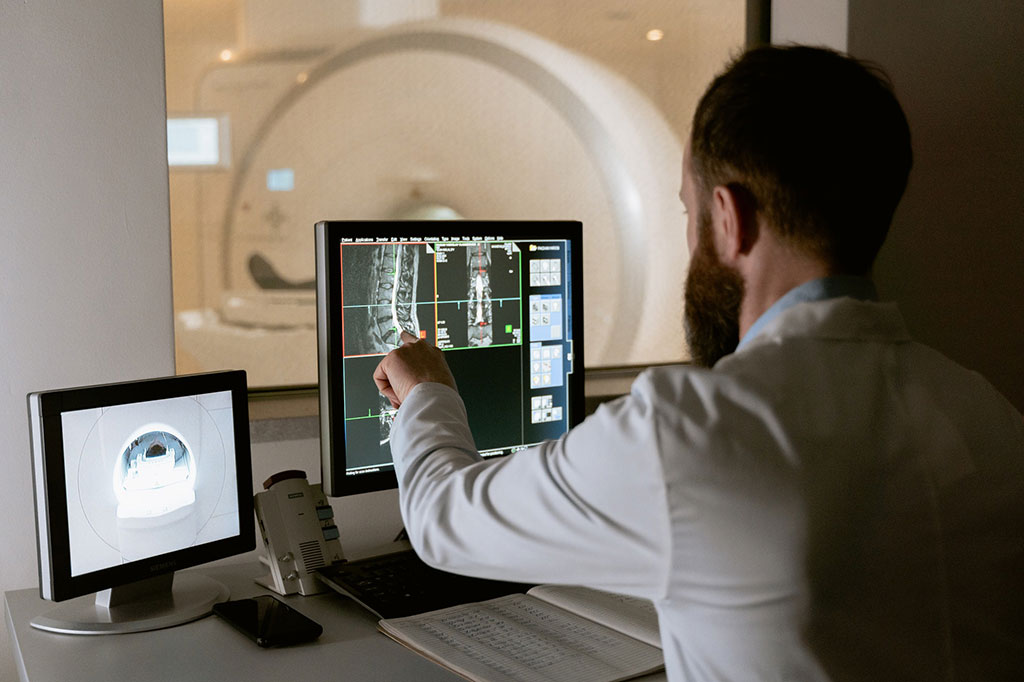
Global AI in Medical Diagnostics Market to Be Driven by Demand for Image Recognition in Radiology
The global artificial intelligence (AI) in medical diagnostics market is expanding with early disease detection being one of its key applications and image recognition becoming a compelling consumer proposition... Read moreIndustry News
view channel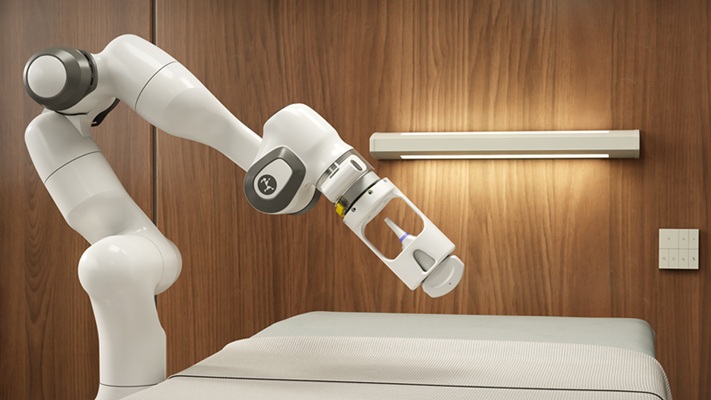
GE HealthCare and NVIDIA Collaboration to Reimagine Diagnostic Imaging
GE HealthCare (Chicago, IL, USA) has entered into a collaboration with NVIDIA (Santa Clara, CA, USA), expanding the existing relationship between the two companies to focus on pioneering innovation in... Read more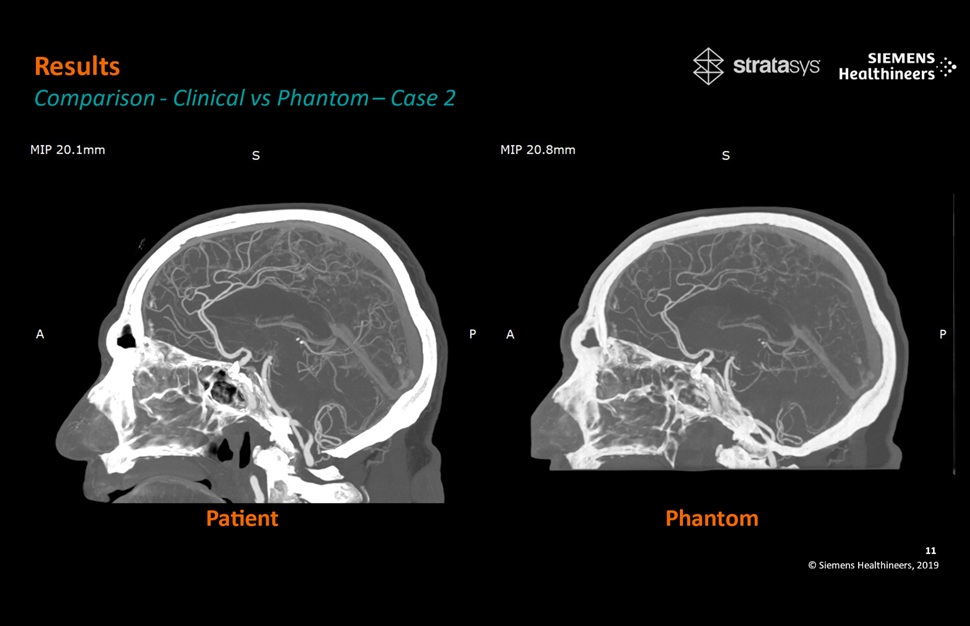
Patient-Specific 3D-Printed Phantoms Transform CT Imaging
New research has highlighted how anatomically precise, patient-specific 3D-printed phantoms are proving to be scalable, cost-effective, and efficient tools in the development of new CT scan algorithms... Read more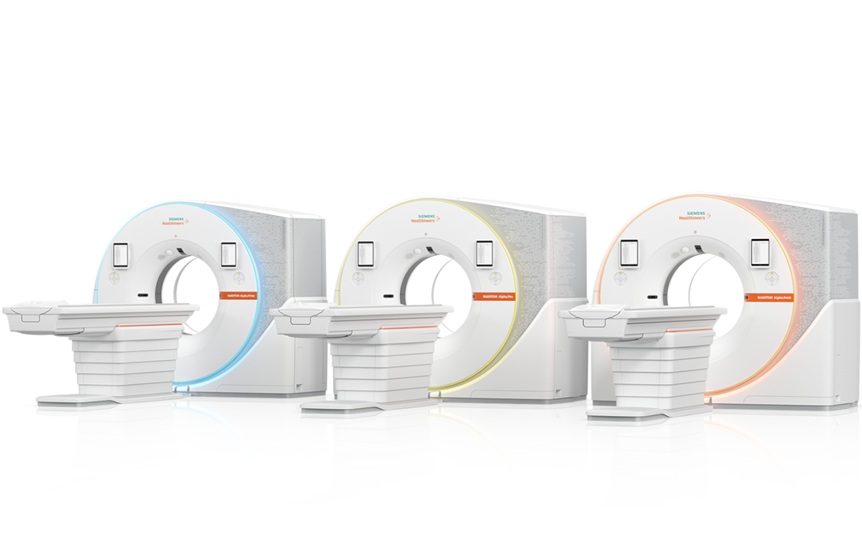
Siemens and Sectra Collaborate on Enhancing Radiology Workflows
Siemens Healthineers (Forchheim, Germany) and Sectra (Linköping, Sweden) have entered into a collaboration aimed at enhancing radiologists' diagnostic capabilities and, in turn, improving patient care... Read more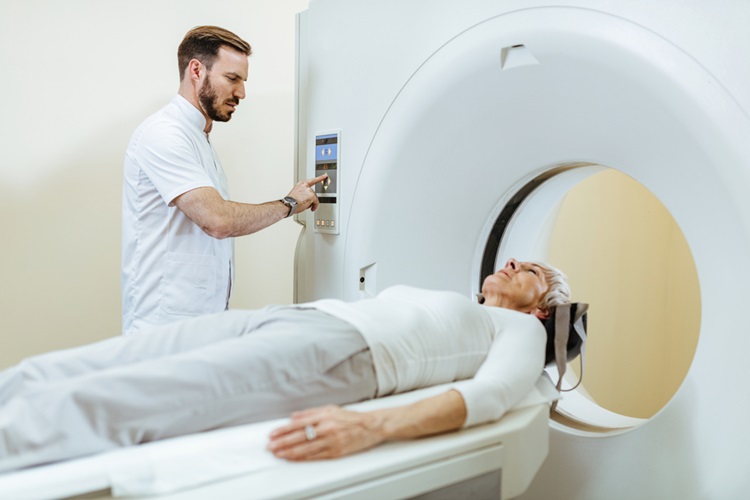