Computational Imaging Technique Creates 3D Model of Mammal Lung Image
By MedImaging International staff writers
Posted on 16 Oct 2012
Scientists are trying to determine more precisely what occurs in the lung’s minuscule, convoluted network of corridors. To accomplish this goal, investigators have created the most detailed, three-dimensional (3D) rendering of the ending of all the pathways in the lung, called the pulmonary acinus. Posted on 16 Oct 2012
The computerized model, derived from mice, authentically imitates the curves in this area, including the direction, length, and angles of the respiratory branches that lead to the air sacs called alveoli. “The imaging and image analysis methods described here provide for branch morphometry at the acinar level that has not been available previously,” the researchers, from the University of Iowa (UI; Iowa City, USA), wrote in their article, published in October 2012 in the online early edition of the Proceedings of the National Academy of Sciences of the United States of America (PNAS).
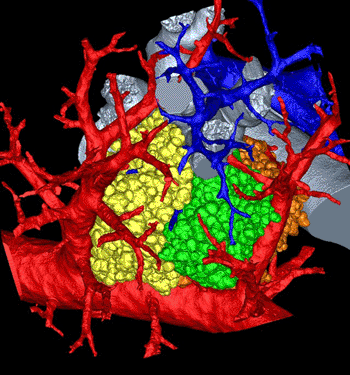
Image: Lung maze modeled in 3D (Photo courtesy of Dragos Vasilescu, University of Iowa and the University of British Columbia).
The model is significant because it can help scientists understand where and how lung diseases emerge as well as the role the pulmonary acinus plays in drug delivery, such as those typically administered with inhalers. “These methods allow us to understand where in the lung periphery disease begins and how it progresses,” said Dr. Eric Hoffman, professor in the departments of radiology, medicine, and biomedical engineering at the UI and corresponding author of the article. “How do gases and inhaled substances get there and do they accumulate in one or another acinus? How do they swirl around and clear out? We just don’t have a complete understanding how that happens.”
For instance, Dr. Hoffman reported that the model could be utilized to determine how smoking-induced emphysema begins. “It has been hypothesized recently that it begins with the loss of peripheral airways rather than the lung air sacs,” he said, mentioning ongoing research by Dr. James Hogg at the University of British Columbia (Vancouver, BC, Canada), who was not involved in this study. It also could provide insights and lead to more effective treatment of chronic obstructive pulmonary disease, which causes irreversible damage to the lung, noted Dr. Dragos Vasilescu, first author on the paper who based his thesis on the research while a graduate student at the UI.
The best, up to now, that lung anatomy specialists such as study co-corresponding author Dr. Ewald Weibel, professor emeritus of anatomy at the University of Bern (Switzerland), could do to study specific areas of a lung was to make measurements in two dimensions or create 3D casts of a lung’s air spaces. The techniques, while offering the first clues into a lung’s composition workings, had their limitations. For instance, they did not directly replicate a lung’s structure in real life, and they could not determine how various parts act together as a whole. However, developments in imaging and computer science have enabled researchers to better study how gases and other inhaled substances act in the lung’s farthest recesses.
In this study, the investigators worked with 22 pulmonary acini taken from young and old mice. They then “reconstructed” the acini based on microcomputed tomography (CT) imaging of scanned lungs in mice and extracted from them. The extracted lungs were preserved in a way that kept the anatomy intact--including the tiny air spaces required for effective imaging. From that, the researchers were able to measure an acinus, estimate the number of acini for each mouse lung, and count the alveoli and measure their surface area.
The mouse lung, in its structure and function, is remarkably similar to the human lung. That means researchers can alter the genetics of a mouse and see how those changes affect the peripheral structure of the lung and its performance. The researchers discovered that mouse alveoli increase in number a long past the two weeks that at least one earlier study had indicated. Dr. Hoffman added that more research is required to determine whether if humans also increase the number of air sacs after a specific, predetermined age.
The researcher’s next objective is to utilize the mouse model to more precisely determine how gases interact with the bloodstream within the acini and the alveoli. “Our imaging and image-analysis methodologies enable new ways to investigate the lung’s structure and can now be used to further investigate the normal healthy-lung anatomy in humans and be used to visualize and assess the pathological changes in animal models of specific structural diseases,” said Dr. Vasilescu, who is a postdoctoral research fellow at the University of British Columbia.
Related Links:
University of Iowa
University of Bern